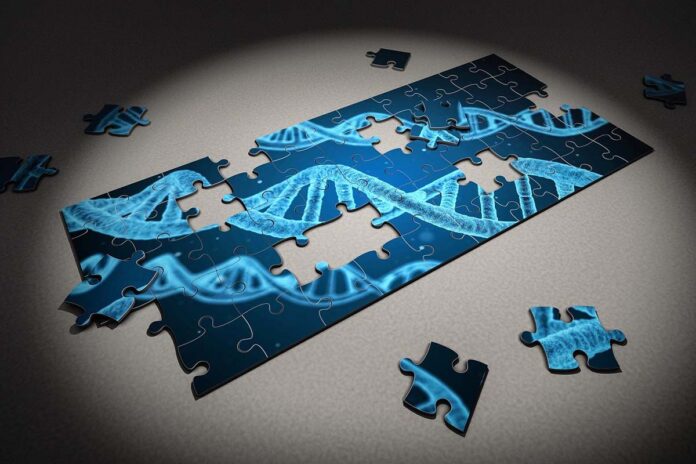
The gut microbiota is a fundamental force influencing diverse aspects of avian physiology. Microbiome studies are at a critical juncture and facing a challenging transition from descriptive studies of association towards mechanistic studies tackling causality. Essential for this transition is a diversity of thinking (chemical and systems biology, metabolism, microbiology, physiology and immunology) and approaches (assays and models).
The gastrointestinal tract, or “gut”, regulates homeostasis of the microbiological, physiological, and physical functions that allows the host to endure infectious and non-infectious stressors that it encounters. Because the gut has the greatest surface area separating the environmentally exposed lumen and the internal subepithelial tissue, it is constantly exposed to infectious and non-infectious stressors making it an active immune organ containing more resident immune cells than any other organ in the host. The gut mucosal immune system, a highly-regulated network of innate and acquired elements, provides a remarkable ability to respond and modify to these extremely diverse encounters. The development of the different divisions of the immune response has corresponded with the acquisition and maintenance of a symbiotic microbiota. The microbiota trains, stimulates, and functionally adjusts the different features of the immune system.
Intestinal immunity
Like the systemic immune system, the mucosal immune system is made up of a network of innate and acquired elements. However, unlike the systemic immune system, the intestinal immune system has two distinct functions: the ability to respond to pathobionts (potential pathogenic microbes), invasive pathogens, and microbial products while also maintaining a state of tolerance to the diverse and beneficial commensal intestinal microbes. Both systems working together through innate immune sensing using pattern recognition receptors (PRRs) on epithelial cells and professional immune cells in the lamina propria (dendritic cells and macrophages), trigger immune pathways resulting in microbial killing and the activation of various acquired immune effector T cells (Th1, Th2, Th17, Treg) all while keeping the resident microbiota in check without generating an overt inflammatory response.
The intestinal innate defenses are characterized by a ‘mucosal firewall’, a system of barriers that separates the lumenal side of the intestine from the subepithelial tissues. The reliability of the mucosal firewall is vital for the interactions between the immune system components and the intestinal contents. The first component of the mucosal firewall is the microbiological barrier where the microbiota live in or at the upper mucus layer. These commensal bacteria function to provide colonization resistance against pathogen colonization, produce metabolites/components that modulate immune signaling, and promote immune homeostasis. The second firewall is the chemical barrier consisting of the mucus overlaying the gut epithelium. The mucus regulates contact between the commensal bacteria and the epithelial cells. This division between the epithelium and commensals is achieved by the activity of the mucus produced by goblet cells in the epithelium, antimicrobial peptides released by the epithelial cells, and mucosal IgA produced by dendritic cells in the intestine. The third component of the firewall is the physical barrier provided by the single cell epithelial cell layer. The intestinal epithelium is a single cell layer that assists the absorption of nutrients while providing a physical barrier that prevents both pathogen invasion and extra- intestinal translocation of commensal microbes. Besides being the primary barrier preventing a microbial breach of the intestine, the epithelial cells should also be considered part of the cellular component of the innate immune response possessing PRRs for sensing microbial-associated molecular patterns (MAMPS), but also capable of producing cytokines and chemokines to drive an inflammatory response against pathogen infection. The final component of the mucosal firewall is the immunological barrier where the professional immune cells (macrophages, dendritic cells, lymphocytes) reside in the lamina propria. Further innate sensing of microbes is conducted by the macrophages and dendritic cells which can present antigens to T cells resulting in the differentiation and activation of various T cell subsets (Th1, Th2, Th17 or Treg). Specialized epithelial cells of the gastrointestinal tract function together with lymphoid, myeloid, and stromal cells to secrete mucus, antimicrobial peptides, IgA, and chemokines that limit direct contact between the epithelium and infectious agents and activate target cells that mediate innate defenses. The importance of these epithelial defense mechanisms is highlighted by the ability of enteric pathogens to target these mechanisms to achieve invasion and dissemination.
Besides being the primary barrier preventing a microbial breach of the intestine, the epithelial cells should also be considered part of the cellular component of the innate immune response possessing PRRs for sensing microbial MAMPS, but also capable of producing cytokines and chemokines to drive an inflammatory response against pathogen infection. The final component of the mucosal firewall is the immunological barrier where the professional immune cells (macrophages, dendritic cells, lymphocytes) reside in the lamina propria. Further innate sensing of microbes is conducted by the macrophages and dendritic cells which can present antigens to T cells resulting in the differentiation and activation of various T cell subsets (Th1, Th2, Th17 or Treg). This infiltration of immune cell in lamina propria is inversely correlated with weight gain, showing that this final component of the mucosa firewall has a metabolic cost for the host that affects animal performance.
Microbiota interactions with immune system
The host-microbiota interaction that affects the host metabolism, immunity and health is exceedingly complex. This crosstalk is mediated by dietary nutrients, host and microbiota metabolites, microbial structural components, as well as antimicrobial compounds. Microbiota growth and anatomical location are regulated by the host through production of non- specific antimicrobial peptides such as defensins, IgA, and miRNAs that regulate bacterial transcripts and bacterial growth.
The commensal microbes in the intestinal tract sense the local environment to induce biochemical pathways to activate bacterial metabolism that allows them to avoid, alter, and/or survive host innate immune killing. Furthermore, some microbial-based molecules can promote specific commensal processes that are beneficial to both host and microbe. Similarly, the host detects the microbes through their production of specific mole ules or components with unique molecular patterns that leads to activation of innate and acquired immune responses. Thus, the adaptation of the commensal bacteria (as well as viruses and fungi) living in the intestine of a host has resulted in a mutually beneficial coexistence for both microbiota and host during homeostasis. The interdependent relationship between host and microbiota pointedly influences the host immune response to induce an immune tolerance to commensal microbes while also maintaining responsiveness to invading pathogens. Altering the intestinal microbial communities disturbs this immune balance and leads to immune dysregulation and susceptibility to diseases.
Sensing of the microbiota by PRRs generates a number of mechanisms that promote the host– microbiota relationship while preventing infection by pathogenic organisms. Microbial signals induce pro-inflammatory cytokines such as IL-23 and IL-1b from macrophages and DCs that then activate IL-17 and IL-22 production by T cells, leading to the production of steady-state physiological inflammation. DCs can carry microbiota antigens to the Peyer’s patches and/or small lymphoid follicles in the avian intestine, where they drive the differentiation of regulatory T cells (Tregs) and Th17 T cells that, in turn, induce the differentiation of IgA- producing plasma B cells that secrete further amounts of IgA.
Microbiota-based metabolites and immunity
The microbiota is directly engaged in maintaining the functional innate immunity of the host. The host immune system consistently senses the intestinal microenvironment to determine the metabolic state and colonization status. In the steady state, the metabolites and/or components of the commensal microbiota are recognized by various PRRs, including toll-like receptors (TLRs) and Nod-like receptors (NLRs), to regulate intestinal epithelial barrier function, cellular lifespan of phagocytes, and induce secretion of antimicrobial peptides and IgA. Furthermore, benefical bacteria ferment dietary fibers to produce small chain fatty acids (SCFA) which stimulate the production of anti-inflammatory cytokines that drives the production of regulatory T cells (Tregs). In addition, the microbiota influences the priming signal of the inflammasome activation that leads to the transcription of IL-6, as well as pro-IL-1β and pro-IL-18. The gut microbiota is involved in maintaining intestinal immune homeostasis by stimulating different arms of the T-cell response. Segmented filamentous bacteria (SFB) are potent promoters of Th17 cells in the intestine; whereas, polysaccharide A from the commensal Bacteroides fragilis stimulates the generation of Tregs (Levy et al., 2017). Alternatively, pattern recognition by TLRs and NLRs can also induce the maintenance of tolerance.
Lastly, it has become readily apparent that the intestinal immune system can also detect the metabolic state of the microbiota by recognition of microbial metabolites via their PRRs. The microbiota, using a number of biochemical pathways, metabolizes both diet- and host-derived metabolites that then influence various components of the intestinal immune system. For example, the microbiota converts non-digestible fibers to SCFA that have a number of anti-inflammatory activities. Dietary tryptophan can be degraded by the microbiota into indoles which promote epithelial cell barrier function. Likewise, the microbiota can metabolize dietary arginine to polyamines that inhibit the production of pro-inflammatory cytokines by macrophages. The microbiota converts primary host-derived hepatic bile acids to secondary bile acids that inhibit pro-inflammatory cytokine secretion by DCs and macrophages. Besides having a repertoire of metabolite sensing receptors, the host has developed immune signaling pathways (inflammasomes) expressed in various intestinal cell subsets (macrophages, DCs, epithelial cells, T cells) that recognize microbial-mediated metabolic activity that can stimulate anti-microbial activity involved in stable colonization of the intestine.
Therefore, there is intimate cross-talk between the microbiota and the host that is steered by metabolite secretion and immune signaling that has critical influence in animal health and disease through multiple physiological functions of the host.
Colonization resistance
The commensal bacteria also provide protection to the host from colonization by exogenous pathogens by a process known as colonization resistance. Two primary mechanisms of colonization resistance have been identified: direct, where the microbiota are in direct competition against pathogen colonization and indirect, where the commensal microbiota stimulate the innate and acquired immune systems as described in the previous sections.
The direct competition of colonization resistance involves multiple processes that include: (a) occupying microbial niches: specific commensal microbes can prevent pathogen colonization to the intestinal mucosa by occupying the niche where a pathogen would normally establish; (b) limiting carbon sources: individual commensals, such as Bacteroides thetatiotaomicron can metabolize fucose (sugar) molecules thereby preventing the availability of this sugar moiety in to certain pathogens in the intestine; (c) siderophore production: some commensals possess the genes for the production and acquisition of the metal ion iron via iron chelators (siderophores) that can uptake iron limiting its availability to pathogens, especially during gut inflammation; (d) production of antimicrobial compounds: some Enterobacteriaceae commensals produce antimicrobial compounds, such as bacteriocins, that target competitor pathogens; (e) contact- dependent delivery of toxins: some commensals can express a type VI secretion system (T6SS), a needle-like injection system that inject toxic proteins into close competitors in a contact-dependent manner.
The indirect mechanisms of colonization resistance against enteric pathogens are mediated by microbiota-stimulated activation of both host innate and acquired immunity. Commensal bacteria can indirectly control pathogen colonization by stimulating of intestinal barrier function and innate immunity as described above. In this case, the commensal bacteria, through the production of metabolites or release of surface components (LPS, peptidoglycans, DNA, etc.) are recognized by the PRRs on the intestinal epithelial and professional immune cells that result in the production and secretion of mucins, secretory IgA (sIgA), and antimicrobial peptides, all of which either increase barrier function of the mucosal firewall or are lethal to pathogens. Furthermore, the commensal microbiota can enhance epithelial barrier function by producing small chain fatty acids (SCFA), such as butyrate, from dietary fibers. T-cell subsets in the intestinal lamina propria are involved in the establishment and the maintenance of colonization resistance. A balanced Thelper/Tregulatory status is generated by diverse populations of commensal bacteria in the intestine For example, segmented filamentous bacteria promote acquired immunity by T cells by stimulating Th17 cells whereas other commensals, such as Clostridium and Bacteroides fragilis , induce the expansion of T regulatory cells that can regulate inflammatory responses through the production of IL-10.
Gut microbiota as an epigenetic regulator of gut function
Epigenetics involves genomic modifications through post-translational and post-transcriptional modification induced by environmental factors, but without modifying the nucleotide sequence of the host cell. Epigenetic mechanisms regulate transcriptional control by external environmental cues such as diet, stress events, disease, infections, and host-microbe cross-talk. Since epigenetic events do not alter the DNA, the epigenomic effects are associated with the attachment of different chemical groups to DNA, histones, and chromatin post-translationally and the epigenetic alterations can persist for several generations. These epigenetic alterations affect both the chromatin structure and serve as recognition elements for proteins with motifs dedicated to binding particular modifications
Since the gut microbiota plays such a pivotal role in poultry metabolism, microbiota-induced epigenetic alterations by dietary nutrients could be a significant environmental factor affecting poultry performance and health. Based on studies in mammals, microbiota –generated metabolites of dietary components can be epigenetic activators of gene expression that modify or inhibit enzymes involved in epigenetic pathways. This can best be exemplified by the production of SCFA (acetate, propionate, butyrate) produced by intestinal microbiota by bacterial fermentation of non- digestible carbohydrates. Butyrate is best known for its beneficial effects on intestinal barrier function, anti-inflammatory activity, and as the primary source of energy to intestinal epithelial cells. Butyrate regulates these biological activities of host gut health by functioning as a histone deacetylase inhibitor (HDAC). Butyrate anti-inflammatory activity is mediated by HDAC suppression of NF-κB in phagocytic cells and dendritic cells, increased production of anti-inflammatory cytokines, and the increased differentiation of naive T cells into T regulatory cells. Other microbial metabolites derived from dietary components, such as nicotinamide adenine dinucleotide (NAD)-dependent deacetylases called surtuins, have been shown to mediate the regulation of epigenetic modifications, including DNA methylation noncoding RNAs and histone modification, in the host intestinal immune-barrier function of mammals. Further research is need to determine whether such gut microbiota metabolite-mediated epigenetic modifications of the immuno-barrier function occur in the poultry intestine.
References are available on request
From the Proceedings of 2019 Animal Nutrition Conference of Canada