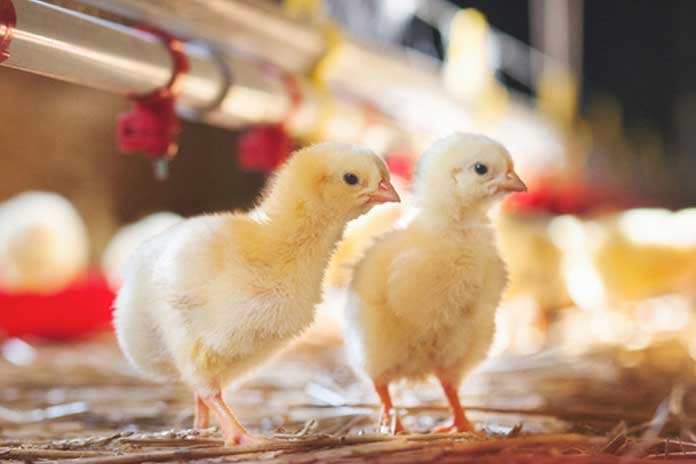
This paper does not address the situations in which the microbiological quality of the water has been compromised from the beginning; rather, this paper addresses how drinking water line systems (DWLS) may contribute to the initiation, prolongation and persistence of a bacteria issue due to certain enabling factors that could potentially facilitate biofilm development.
Production animal facilities are facing new challenges in how to produce the same or greater amounts of animal protein without the use of biosecurity protocols that were previously available. One of the examples is the use of antibiotics in feed or water for the prevention or reduction of pathogenic bacteria. With this picture in mind, production animal managers and servicemen are reviewing the basic elements in their farms, such as water and its role as a potential source and/or persistence factor for bacteria.
Biofilm progressive development emerges over an inert surface such as the internal sides of the DWLS that are used inside a broiler barn. This substance proliferation will enable an appropriate media for bacteria and another contaminants proliferation. These new contaminants will become residents and sometime could multiply inside those surfaces and maintain protected inside those layers of polymeric substances. These new layers and contaminants on the new inner surface will limit the action of chemicals, such as the disinfectants.
Introduction
There are many factors that may enable or facilitate biofilm development such as:
Corrosiveness
This is one of the major contributors to biofilm development. Studies have shown that biofilms developed more quickly on iron pipe surfaces than on plastic PVC pipes. Biofilms are difficult and practically impossible to eliminate from the surfaces in drinking water distribution systems due to the protection offered by the microbial self-produced extracellular polymeric substance (EPS) matrix. This matrix protects microorganisms within biofilms from external adverse factors and fluctuations, including chemical disinfection.
Chemicals
Besides the susceptibility of the material of the water pipe itself, another contributor could be the type of routine/daily products put through the drinking water line systems (DLWS) with the intention of reducing water bacteria contamination.
Some chemistries could prompt higher and faster corrosive effects, such as oxidative chemistries on iron or copper-based pipe surfaces.
Chlorine residues
This includes levels that are insufficient for eliminating bacteria but are sufficient for creating a zone of tolerance that allows the persistence of bacteria such as the coliform. A water line system could experience coliform occurrences even when free chlorine residues average between 2 and 2.5 mg/L. Use of m-T7 medium, a technique that recovers injured bacteria, has shown coliform occurrence rates ranging between 10% and 40% — even during months when coliforms were not recovered on the standard m-Endo medium.
Other combined factors
The penetration of free chlorine into a biofilm has been modeled and shown to be limited by its fast reaction rate. Essentially, free chlorine is consumed by reaction-diffusion interaction with cellular biomass before it can react with the bacterial components of the film. Stewart and colleagues demonstrated that free chlorine effectively did not penetrate alginate beads containing bacterial cells. The corrosion of iron pipes can influence the effectiveness of chlorine-based disinfectants for the inactivation of biofilm bacteria. Therefore, the choice of pipe material and the accumulation of corrosion products can dramatically impact the ability to control the effects of biofilms in DWLS. This variation in corrosion rates is important because the corrosion products react with residual chlorine, preventing the biocide from penetrating the biofilm and controlling bacterial growth. The use of chlorine-based disinfectants under such conditions are very common in today’s animal production facilities.
And finally, certain products added into the water system
Some additives and supplements that are used through DWLS may facilitate the development of biofilms because of their adjuvants, vehicles, pH fluctuations or other factors of inherent to its formulations. The frequent administration of these types of products by servicemen and production animal caregivers is another factor that might worsen or contribute to the building up of — or the predisposition towards — biofilms in water lines.
Biofilm-associated populations and characteristics
Under field conditions, some of the biofilms reported have been associated with Gram-negative bacilli, Pseudomonas, Acinetobacter spp., Klebsiella spp., and others. Parasites like Cryptosporidium could be trapped in biofilms. Although viruses and Cryptosporidium do not grow in biofilm, they can attach to biofilms after a contamination event. Therefore, it is important to thoroughly flush the distribution system to remove these organisms following a contamination event. An intensive monitoring and sampling study was performed in England during the seasons of 2012 and 2013 to accumulate information that may show patterns of seasonality and other potential influential factors over the microbial dynamics inside DWLS under chlorinated treatments. Samples were collected before and during flushing operations during a four months’ interval, 5 litters each. The detailed microbial analysis was performed using 16S rRNA Illumina MiSeq sequencing. This microbial profile (Graph 1) showed a higher tendency for biofilm formation on cast iron water line surfaces compared to plastic or PVC, as well as some evidence of a potential favorable effect of the warm seasons. It is important to highlight the Clostridia findings under this particular study, as Clostridia has historically been one of the most critical bacteria to deal with in poultry facilities; because of its difficulty to be removed from poultry farms and its increasing deleterious effect under reduced or none-antibiotic production programs. Chlorine residue levels correlated negatively with Bacteroidetes and Sphingobacteriia and correlated positively with Clostridia. The positive correlation between Clostridia and chlorine can be explained by the known ability of this microorganism to form spores that are very resistant to chlorine and other disinfectants. These results confirm that biofilms provide an environment in which bacteria are protected from chlorine residue. An example of the inefficiency of chlorine in controlling biofilms is the high presence of mycobacteria in the plastic pipe samples.
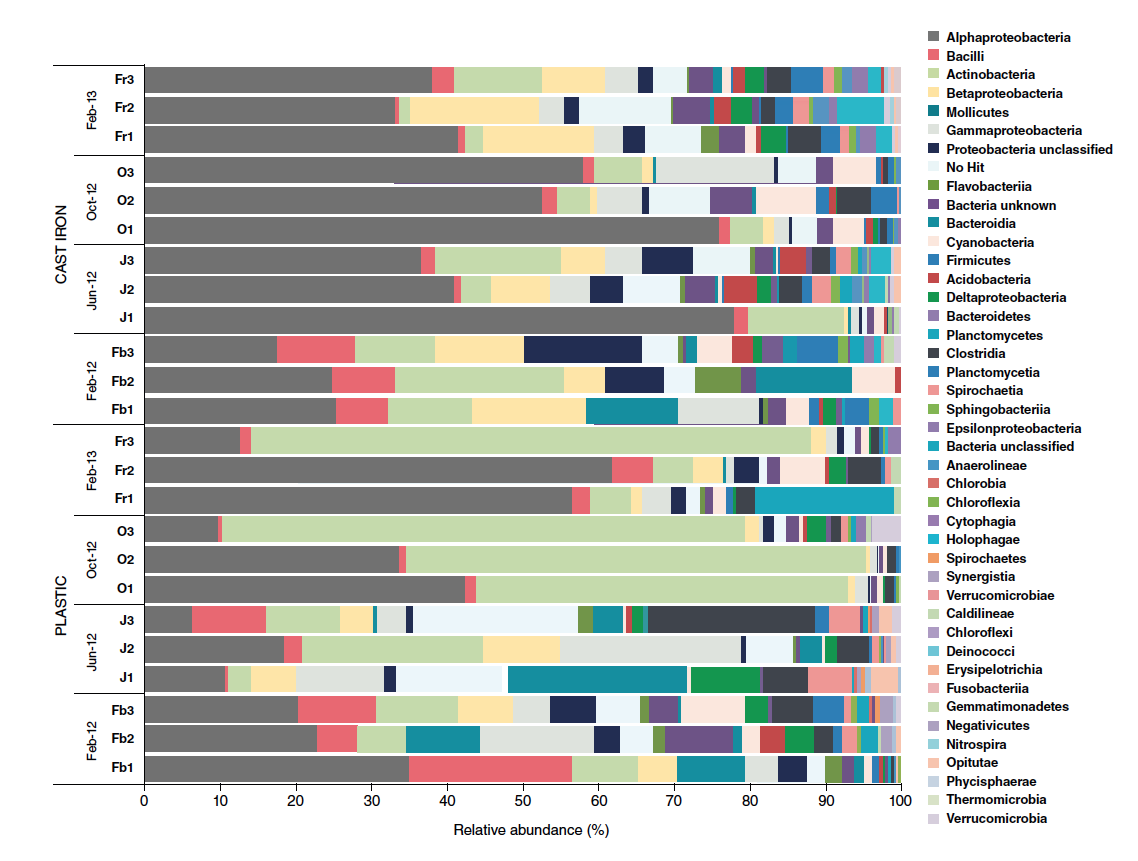
Biofilm control, management and prevention
These processes could be perform using various approaches, such as: mechanical action, the first line of defense for water lines is to perform systematic flushing of their internal surfaces as performed in other industries, such as clean-in-place (CIP) protocols. Flushing the water lines should be one of the routine protocols performed in order to reduce the slime that may develop between flocks, and also in long-living flocks, with modified protocols to reduce the risk of nipple drinkers clogging. Doing so avoids potential water restrictions, potential egg drops and/or negative effects in performance. The temperature of the water used to apply and flush the system could be also a potential beneficial component, because water temperatures between 20 °C and 32 °C could facilitate surfactant action in the surface to affect the superficial tension and help to expose and break the biofilm community. Second through the use of detergents; acid detergents have a powerful descaling ability in the internal surface of the water line. The properties of a lower pH solution could help to reduce the superficial tension of the biofilm and enable the degradation of the bio-slime material, thus making bacteria more susceptible to the environmental conditions and any chemicals added within the detergent formulations. Third by specific chemicals; some specific chemical principles that work for the immediate and gradual removal of biofilm formations, such as the combination of stabilized hydrogen peroxide and silver nitrate. This chemistry could be used in terminal disinfection conditions at the end of flock removal. Other chemical combinations, such as peracetic acid- based products plus stabilized hydrogen peroxide, have been demonstrated as highly effective in working against the removal of scale and biofilms – at the same time lowering the bacteria log as disinfection properties. These chemical combinations could be used in the removal strategy of biosecurity interventions that address the reduction of the current layers of biofilm that have been developed. There are other chemicals, such as chlorine dioxide, that could be of use in constant dose controls, with on-demand usage based on a pre-settled dose calibration protocol. The precursor of chlorine dioxide is activated with an acid-based product delivered into the DWLS, followed by a change of water pressure when animals are drinking water. So, the activation will occur just when it is needed: on-demand. This final product will help to control and manage biofilm formation. Removing biofilm and reducing biofilm formation helps to manage the negative impact on the taste and odor of the water. Additionally, chlorine dioxide binds mineral elements, such as iron and manganese, that could cause taste and odor issues. These minerals have also been noted as fundamental precursors for bacteria growth. We are not going to discuss in this paper the role of chlorine dioxide as a disinfectant, but it has been demonstrated and reported in previous literature
Materials and methods
Chemical products for water intervention
Installation of a hydrogen peroxide/silver nitrate dosage system in the water lines in the farms for study 1 and 2. Installation of a chlorine dioxide (ClO2) on dosage demand water line system with two pumps to regulate ClO2 activation by an acid for study 3.
Measuring protocols and metrics
For the three-studies water consumption was evaluated, performance parameters, such as average body weight, feed conversion and mortality, and for study 3 percent of egg production and peak persistence was evaluated. For study 1 a comparison of antibiotic usage was done versus the historical of the same farms with previous flocks. For study 2, a luminometer based equipment that detects adenosine triphosphate (ATP) was used to assess the potential amount of biofilm in 100cc of sampled water before and after water treatment. For study 3 a conductivity tool was used to evaluate the consistence of oxidation reduction potential (ORP) units to try to make sure that the water system was always between 600-800 milivolts (mv). A CHEMetrics instrument was used as well to evaluate the free ClO2 available in the water line at the end of the drinking line, the goal was to achieve an average concentration between 1 to 2 PPM of free ClO2. The pH was also measure in a weekly basis, to verify any major variations.
Results
Biofilm experiences
Study 1: Reducing antibiotic usage
The performance records of one poultry complex in the USA were collected between 2013 and 2015. Before installing a hydrogen peroxide/silver nitrate protocol for biofilm and water treatment, the farm was experiencing between 19 and 25 treated flocks per year, mainly due to enteric, respiratory and infectious processes associated with E. coli and other opportunistic bacteria. For the year 2015, farms from the same company and same broiler complex had to treat just six flocks from the entire year around. A very different bacteriological picture was achieved after the use of this protocol as part of the program to reduce antibiotic usage in the flocks (See summary of results in Graph 2).
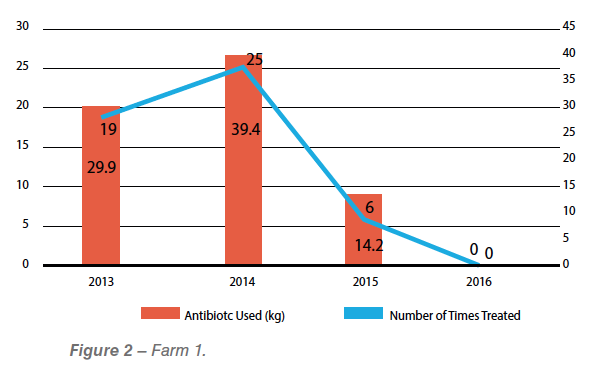
Study 2: Reducing biofilm in water lines
Seven different farms were involved in another study. Water samples were collected at the end of the water line from each of the seven farms before and after treating the water line with a hydrogen peroxide/silver nitrate protocol. Five of the seven farms showed lower levels of biofilm after the treatment. The levels of biofilm were measured using a luminescent-based technology to detect adenosine triphosphate (ATP). The use of ATP luminescent readers to support sampling of hard surfaces and/or water that may contain biofilm is very reliable, and it had shown high correlation with a lack of hygiene on surfaces.The results are expressed in Relative Luminescent Units (RLU) the higher the units, the higher the presence of contaminants, such as biofilm (See summary of results in Graph 3).
Study 3: Improving below-average farms
A commercial layer facility in the U.S. that started the adoption of water line treatment protocols between 2016 and 2017 experienced positive changes in different performance index. Some of the below- average company farms that struggled during the peak of production and the weeks of persistence of production (because of the numerous additional stress factors) were included as field treated populations. These farms were the first to show a dramatic change during those approximate 20 weeks of critical egg production. These farms previously fell 3% to 4% below the average of egg production from overall company-accumulated parameters. During this critical period of 20 weeks, these farms were reporting an increased mortality associated with peritonitis and an overall increase of respiratory issues from their usual figures. Previous records of water consumption were compared between 2016 and 2017 in the same farms for the same time of the year, and it was found that water consumption was higher for those historical below-average farms, demonstrating that the palatability of the water was affected before by the scale and biofilm contents in the water lines and the water itself. The production performance during those 20 weeks became consistent and comparable to the average best-performing farms within the company, including average egg-mass and a reduced prevalence of peritonitis and respiratory issues associated with E. coli (personal communication, 2018).
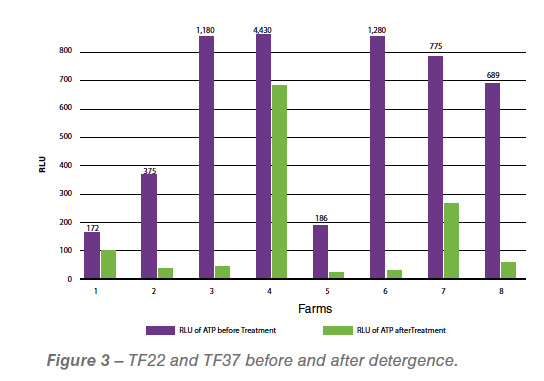
Discussion
Certain management practices currently used for drinking water line systems may lead to the increased risk of developing biofilms, and the problem can persist into the farm and contribute to the growth of resident bacteria. Biofilm formation could help to maintain bacteria associated with this type of community formation, creating a cycle of potential contamination for the entire production site.
Traditional disinfection practices have not demonstrated an efficient performance for the prevention and/or removal of biofilm in drinking water line systems. But new chemistries, like the ones mentioned in this paper, could prove to be part of more effective interventions and protocol practices for animal production facilities.
In addressing these types of challenges from drinking water line systems, there will be a high possibility of a reduced need for the use of antibiotics, because addressing these challenges should lower the level of bacteria within the biological animal production systems.
In commercial layer farms, as well as turkey farms, certain bacteria profiles may serve as indicators of water lines that face tough issues in regard to biofilm. An example of this is the frequency of finding Ornithobacterium rhinotracheale.
References are available on request
From the Proceedings of the 68th Western Poultry Disease Conference